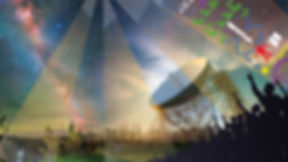




Communicating by waves
Following the prediction of “electromagnetic waves” by James Clerk Maxwell, Heinrich Hertz realised the first radio transmission in 1886, and in 1901, Guglielmo Marconi sent the first radio message across the Atlantic Ocean from England to Canada. The underlying key technology has since emerged to be used commonly for wireless communications including radio and TV stations, mobile phones, and computer networks, with base stations no longer being restricted to Earth, but involving satellites as well. On 21 July 1969, we saw a live broadcast as Neil Armstrong made his steps on the Moon.

Fig. 1: Common use of radio technology for communication across the globe and beyond.
Frequency and wavelength
An electromagnetic wave can be understood as electric and magnetic fields of oscillating strength, where the maximum travels with the speed of light, which is about 300,000 km/s in vacuum. In fact, unlike sound waves, electromagnetic waves do not need a medium (such as air) to propagate. The rate of oscillation is called the frequency, and is usually measured in Hz (Hertz), where 1 Hz means one oscillation per second. If we divide the propagation speed of the wave by the frequency, we find the wavelength, which is the spatial distance between subsequent maxima. Radio frequencies range from 3 kHz to 300 Ghz, while radio wavelengths range from 1 mm to 100 km. Light is another form of electromagnetic waves, however with a much smaller wavelength, between about 400 nm and 800 nm.
Wave modulation and signals
In order to transmit information by means of radio waves, we modify a carrier wave by altering its strength (amplitude) or its frequency, known as amplitude modulation (AM) or frequency modulation (FM), respectively. The information content is in the modulating waveform rather than in the carrier.
The modulating waveform can represent either an analogue or a digital signal, where the latter can in particular be realised as a rectangular wave with the amplitude switching between two discrete values at a given bit rate. Every waveform can be considered a superposition of oscillations of a given frequency, with its spectrum showing the strength of these elementary components as function of frequency. This means that if we scan a radio broadcast, we see a strong carrier with narrow-range sidebands that constitute the transmitted information.

Fig. 2: Amplitude and frequency modulation of a carrier wave by a signal.
The advent of radioastronomy
During the second world war, huge advances were made in the field of radio science. In particular, powerful transmitters began to appear, in order to support powerful military (and later civilian) radar systems. Much of the technology development was carried out by young physicists in the UK, USA, Australia and Germany. After the war had ended, these physicists returned to their university appointments to restart their previous academic research programmes. Many began to use the new radio technology to conduct observations of the sky, and the first large radio telescopes began to appear in the mid-1950s. Sir Bernard Lovell, who had pioneered airborne radar during the war, constructed a huge 250-ft radio telescope, located at Jodrell Bank, near Manchester.
Radio and SETI
In 1959, Giuseppe Cocconi & Philip Morrison realised that these large radio telescopes were sensitive enough for radio transmissions to be sent and received across enormous distances. For the first time, it became possible to think about the Search for Extra-Terrestrial Intelligence (SETI) via the detection of artificial radio signals transmitted by other technical civilisations distributed across the Milky Way.
Radio signals are particularly favoured for SETI surveys because they are not absorbed by the large quantities of dust and gas that lie between the stars, quite unlike optical signals. In addition, the low energies involved in producing radio waves means although they can travel enormous distances unimpeded, they are easy and cheap to generate, including in particular very powerful narrow-band signals. It also transpires that the radio sky is also rather “dark”, with natural radio emission from Milky Way stars occurring very rarely. Moreover, the narrow-band spectrum of our terrestrial radiocommunications can be easily distinguished from the broad-band spectra that arise from natural (non-technological) emission from stars or galaxies.
SETI surveys - looking for a needle in the haystack
There is no doubt about, detecting a SETI signal is going to be difficult. We have no idea where the signals will come from on the sky, when they will be transmitted, and at what frequency will they be broadcast. For a narrow-band transmission, we also need to consider a drift in the central frequency of the signal due to the continuously varying relative velocity of the receiver on Earth and the extra-terrestrial transmitter. While we can try and make some guesses on some of these matters, a great deal of parameter space needs to be searched through – it’s very much like looking for a needle in a haystack!
An explorative approach to SETI is to analyse all data that are acquired by radiotelescopes. Artificial Intelligence can be rapidly trained to recognise new signals and thereby efficiently eliminate most human-made signals, so that scientists can focus their efforts on signals that are potentially extra-terrestrial.
SETI signals and the number of intelligent civilisations
The number of SETI signals we can expect to detect depends on two key factors: the rate at which communicating civilisations arise, and the time span over which they last and keep communicating. While the first factor encompasses all of our evolutionary history, the second factor is about sustainability and a glimpse into our future. Notably, the number of detections does not tell us whether there are many civilisations that last for a short time, or rather few that last for a long time. Given that it is easier to detect other civilisations nearby rather than far away, one of the most relevant big unknowns is how close our nearest neighbours are.
SETI - then and now...
Frank Drake was the first person to perform a SETI survey of nearby stars in the early 1960s. His “Drake Equation”, breaking down the chances of success into a series of factors, set a first agenda for framing the search for life beyond Earth.
Various other SETI surveys followed. Today, the Breakthrough Listen initiative (funded by entrepreneur Mr. Yuri Milner) is conducting the first large, and systematic SETI surveys using telescopes all over the world – USA, Australia, South Africa and the UK. The Breakthrough Listen surveys will be many orders of magnitude more capable than the early surveys of the 1960s. In one day, Breakthrough Listen will survey as much parameter space as all previous SETI surveys added together. The prospects of a SETI detection have never been better!

Fig. 3: Some of the telescopes involved in the Breakthrough Listen SETI surveys and follow-up observations. The latter will also involve the e-MERLIN telescope network located in the UK, including the iconic 250-ft Lovell Telescope at Jodrell Bank.